Electron spin
What is electron spin?
Electron spin is a property of electrons that manifests itself as a magnetic force in a magnetic field. It is difficult to say what the electron spin really is. In the so-called Stern-Gerlach experiment, it can be shown that there is an effect of force between magnetic fields and electrons that can only be explained by electron spin. Today, many magnetic properties of matter, such as para- and ferromagnetism, can be explained by electron spin.Table of Contents
Many elementary particles have a spin.
While it is easy to picture mass or charge as properties of elementary particles, it is more difficult with spin.
An electron, for example, has a mass of 9,1•10-31
kg and a charge of -1,6•10-19
C.
The spin is often only given as a quantum number and, depending on the orientation, is +1/2 (spin up) or -1/2 (spin down) for electrons.
According to quantum theory, spin is a similar property to angular momentum.
In mechanics, for example, angular momentum is caused by circular motion.
In contrast to a simple circular motion, however, spin is a fundamentally unchangeable property.
Although the direction of spin can be changed, it cannot be reduced or increased.
Observation of electron spin in the Stern-Gerlach experiment
The spin was observed experimentally through the Stern-Gerlach experiment. In this experiment, silver atoms were sent through a strong inhomogeneous magnetic field.
In the Stern-Gerlach experiment, silver atoms are vaporised in a furnace.
The atoms (shown in grey) then fly out of an opening into a strong and inhomogeneous magnetic field (hinted at in red).
An inhomogeneous magnetic field has very different strengths at different points in space.
As a result, a force acts on magnetic moments, which leads to a deflection of the atoms on their trajectory.
Since the silver atoms do not have any significant magnetic moments (apart from the electron spin), it was expected before carrying out the experiment that the atoms would only be deflected slightly and not in certain preferential directions but in a continuous distribution.
However, two discrete spots were observed on the observation screen set up behind the magnetic field.
Consequently, there must be a magnetic moment of the silver atoms that has exactly 2 possible settings and was previously unknown.
This led to the discovery of the electron spin.
Although it had been assumed before the Stern-Gerlach experiment that silver atoms had no magnetic moment,
it was observed that the silver atoms were deflected in the magnetic field, i.e.
a magnetic force
acts on the silver atoms.
In the Stern-Gerlach experiment, half of the atoms are deflected in a certain direction, while the other half of the atoms are deflected in exactly the opposite direction.
The Stern-Gerlach experiment was able to prove that the atoms do have a magnetic moment upon which a force acts in the inhomogeneous magnetic field. In addition, this magnetic moment must have two possible orientation directions. Physicists, therefore, required a property of the electrons that would explain the observed magnetic force effect. This property was called electron spin. Prior to the Stern-Gerlach experiment, electron spin was not known, as there was no reason to necessitate such an additional spin property of electrons. Only the demonstration of the Stern-Gerlach experiment showed that there must be such a previously unknown property. The electron spin was thus discovered due to its magnetic moment and then incorporated into quantum theory to describe electrons. It was postulated that it can only have two orientation directions, namely spin up or spin down.
The spin of a particle has significant consequences for the motion and location of the particle. According to the Pauli principle, two electrons in an atom cannot be identical in all their properties. During the development of the periodic table, it was already established that, in a state with the same energy and the same angular momentum, there can always be exactly 2 electrons in an atom. However, these 2 electrons must then differ in their spin. Both electrons have oppositely orientated spins. The magnetic moments of these spins then offset each other. The electrons are "paired". If atoms only have such paired electrons, the total spin of the atoms, which is predominantly determined by the electron spin, is approximately zero. The atoms then have no resulting total spin that could align itself in an external magnetic field and thus behave diamagnetically.
However, the silver atoms in the Stern-Gerlach experiment have a so-called unpaired electron. Their resulting total spin corresponds approximately to the spin of this electron. In the case of oxygen, for example, even 2 electrons are unpaired and can align themselves in the magnetic field. In both cases, we speak of paramagnets. Paramagnets align their spins in an external magnetic field and are therefore attracted.

The illustration shows a schematic view of the electron configuration of helium (diamagnetic) with a resulting total spin of 0 on the left and the electron configuration of triplet oxygen with a resulting total spin of 1 on the right.
The spins of the electrons are shown as arrows, which symbolise the associated magnetic moment.
In triplet oxygen, 2 electron spins are unpaired.
The oxygen is paramagnetic.
The model shown is not exact and is for illustrative purposes only.
In some materials, there is a strong interaction, the so-called exchange interaction,
between the aligned electron spins.
The exchange interaction is also caused by the Pauli principle.
If it is greater than the thermal energy of the electrons, the material behaves in a ferromagnetic
way in an external magnetic field.
The electron spins then remain aligned even after the external magnetic field is switched off, which can be measured as remanence, i.e.
as a residual magnetisation.
Today, it is now known that protons and neutrons also carry a spin, but its magnetic effect is a thousand times smaller than that of electrons. The spin of protons and neutrons is formed by the spin of the elementary particles, i.e. the quarks, which make up the protons and neutrons.
According to the elementary particle model, all elementary particles have spins of different sizes. The spin can always be detected via its magnetic moment. As stated by Maxwell's equations, the laws of electricity and magnetism, a magnetic moment is always created by a current, i.e. electron motion.
The electron spin concept: no satisfactory answer to date
What electron spin really is has not yet been satisfactorily explained. In the past, spin was thought of as the rotation of spherical elementary particles around their own axis.However, it leads to contradictions to imagine the spin of electrons as a rotation around their own axis. This is already inconsistent because electrons are not simply small charged spheres, as was previously assumed. Rather, electrons have certain properties of waves with a certain wavelength and certain characteristics of spheres, such as the fact that electrons can be "knocked out" of an atom.
From a physics point of view, the problem of electron spins has been sufficiently addressed if the electron spin is identified with the measurable magnetic moment or if reference is made to spin degrees of freedom in modern mathematical theories.
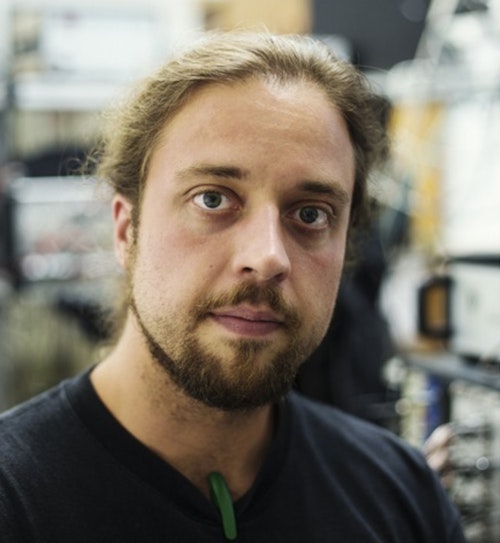
Author:
Dr Franz-Josef Schmitt
Dr Franz-Josef Schmitt is a physicist and academic director of the advanced practicum in physics at Martin Luther University Halle-Wittenberg. He worked at the Technical University from 2011-2019, heading various teaching projects and the chemistry project laboratory. His research focus is time-resolved fluorescence spectroscopy in biologically active macromolecules. He is also the Managing Director of Sensoik Technologies GmbH.
Dr Franz-Josef Schmitt
Dr Franz-Josef Schmitt is a physicist and academic director of the advanced practicum in physics at Martin Luther University Halle-Wittenberg. He worked at the Technical University from 2011-2019, heading various teaching projects and the chemistry project laboratory. His research focus is time-resolved fluorescence spectroscopy in biologically active macromolecules. He is also the Managing Director of Sensoik Technologies GmbH.
The copyright for all content in this compendium (text, photos, illustrations, etc.) remains with the author, Franz-Josef Schmitt. The exclusive rights of use for this work remain with Webcraft GmbH, Switzerland (as the operator of supermagnete.gr). Without the explicit permission of Webcraft GmbH, the contents of this compendium may neither be copied nor used for any other purpose. Suggestions to improve or praise for the quality of the work should be sent via e-mail to
[email protected]
© 2008-2024 Webcraft GmbH
© 2008-2024 Webcraft GmbH